The Google Sycamore quantum computer has captured the world’s attention with its revolutionary approach to computing. By leveraging principles of quantum mechanics, it promises to solve complex problems that are far beyond the reach of traditional computers. This article delves into the details of Sycamore, how it works, the implications of its capabilities, and the challenges that still lie ahead.
What Is Quantum Computing?
To understand Sycamore’s impact, we first need to grasp the concept of quantum computing.
How Traditional Computers Work
Traditional computers, from smartphones to supercomputers, process information in binary bits. These bits can only be in one of two states: 0 or 1. This binary system is the foundation of all modern computing. Every calculation, software function, or internet search relies on the manipulation of these binary bits.
How Quantum Computers Are Different
Quantum computers operate using quantum bits, or qubits, which follow the rules of quantum mechanics—a branch of physics that describes how subatomic particles behave. Qubits are fundamentally different from traditional bits in two important ways:
- Superposition: Unlike traditional bits, which can only be in a state of 0 or 1, qubits can be in a state of 0, 1, or both simultaneously. This phenomenon, called superposition, allows quantum computers to process a vast amount of data in parallel.
- Entanglement: When qubits become entangled, the state of one qubit is dependent on the state of another, even if they are separated by large distances. This property enables quantum computers to solve problems more efficiently by sharing information between qubits instantaneously.
Why Do We Need Quantum Computers?
Quantum computers excel at solving problems that involve many variables and complex interactions, whereas traditional computers would take an impractical amount of time. Fields like cryptography, drug discovery, and climate modeling all involve problems that are extremely challenging for even the best supercomputers. Quantum computers could potentially revolutionize these fields by finding solutions in minutes that would otherwise take thousands of years.
Introducing Google Sycamore Quantum Computer:
The processor of the Google Sycamore quantum computer is designed to achieve something called quantum supremacy—the point at which a quantum computer can solve a problem that is virtually unsolvable by classical computers within a reasonable time frame.
What Is Quantum Supremacy?
Quantum supremacy refers to the moment when a quantum computer performs a calculation that no traditional supercomputer could achieve in a realistic timeframe. It doesn’t mean that quantum computers will instantly replace classical ones, but it signifies a landmark achievement showing quantum technology’s potential.
The Sycamore Processor: How It’s Built
The Sycamore quantum processor consists of 54 qubits, arranged in a two-dimensional grid. During its breakthrough experiment, 53 qubits were operational, which was still sufficient to demonstrate quantum supremacy. These qubits are made from superconducting materials and are kept at extremely low temperatures, close to absolute zero, to ensure their stability and reduce interference from outside sources.
The Breakthrough Experiment
In 2019, Google announced that Sycamore had achieved quantum supremacy. The experiment involved performing a complex calculation known as random circuit sampling, which involves verifying the output of randomly generated quantum circuits.
- The Task: The Sycamore processor was programmed to sample the output of a complex quantum circuit with thousands of operations.
- The Achievement: Sycamore completed the task in 200 seconds (just over three minutes).
- Traditional Comparison: IBM’s Summit, the world’s most powerful classical supercomputer at that time, would have taken around 10,000 years to perform the same calculation. This stark difference highlighted the potential of quantum computing to solve certain problems exponentially faster than traditional methods.
The Technology Behind Sycamore:
To understand Sycamore’s capabilities, we need to explore the core principles of quantum mechanics that make quantum computing possible.
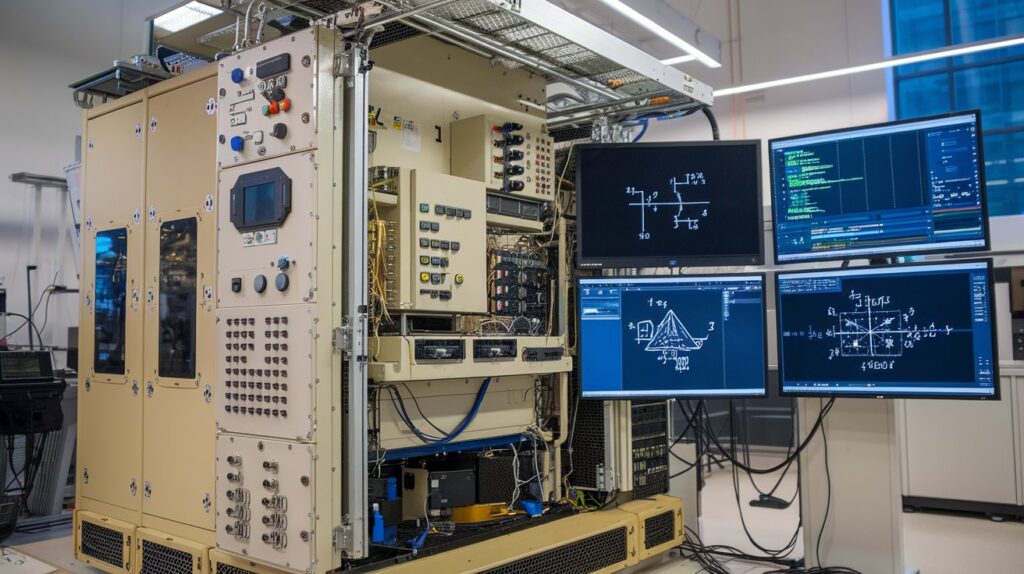
1. Superposition: Multiple States at Once
Superposition allows qubits to exist in multiple states simultaneously. Imagine a bit as a coin that can either show heads (0) or tails (1). In a traditional computer, the coin must land on heads or tails before any calculations can be made. In a quantum computer, the coin can exist in a state of spinning where it represents both heads and tails at the same time. This ability to exist in multiple states allows quantum computers to explore many possible solutions at once.
2. Entanglement: Linked Qubits
Entanglement is a quantum phenomenon where the state of one qubit is linked to the state of another, regardless of the distance between them. Changing the state of one qubit instantly affects the state of the other. This interconnectedness allows quantum computers to solve complex problems much faster than traditional computers by sharing information between qubits instantaneously.
3. Quantum Interference: Enhancing the Right Answers
Quantum algorithms take advantage of interference to amplify correct answers and diminish incorrect ones. It’s akin to finding the right path through a maze by illuminating the correct routes and darkening the dead ends. This process helps quantum computers zero in on the correct solutions more efficiently.
Challenges Facing Sycamore and Quantum Computing
While Sycamore’s achievement is impressive, there are still several significant hurdles that must be overcome to make quantum computing practical for everyday use.
1. Qubit Stability and Decoherence
Qubits are incredibly sensitive to their surroundings, such as temperature changes and electromagnetic interference. These environmental factors can cause qubits to lose their quantum state, a problem known as decoherence. To keep qubits stable long enough to perform calculations, quantum computers must operate at extremely cold temperatures (near absolute zero).
2. Error Rates and Correction
Quantum computers currently suffer from high error rates due to qubit instability. Quantum error correction is an ongoing field of research that aims to solve this issue by using multiple physical qubits to form a single “logical qubit.” This logical qubit is more stable and can withstand errors better, but the approach requires many more qubits than the current technology allows.
3. Scaling Up the Number of Qubits
To solve practical and commercially relevant problems, quantum computers will need to have thousands, if not millions, of stable qubits. The challenge is not just building more qubits but also maintaining their stability and reducing error rates as more qubits are added. Scaling up is one of the most significant hurdles for the future of quantum computing.
You must visit: Salesforce Service Cloud: Features, Benefits, and Future Prospects
Why Quantum Computing Matters:
If these challenges can be overcome, the possibilities for quantum computing are immense.
1. Drug Discovery and Molecular Simulations
Quantum computers could revolutionize the pharmaceutical industry by accurately simulating the behavior of molecules and chemical reactions. Traditional computers struggle with these simulations because the number of variables involved is enormous. Quantum computing could help researchers develop new drugs and treatments much more quickly.
2. Cryptography and Data Security
Current encryption methods rely on the difficulty of solving certain mathematical problems, like factoring large numbers. Quantum computers, with their immense processing power, could solve these problems in a fraction of the time, potentially making existing encryption methods obsolete. However, they could also create new, more secure encryption techniques that are resistant to quantum attacks.
3. Optimization Problems
Quantum computers could solve optimization problems far more efficiently than traditional computers. For example, finding the best delivery routes for logistics companies or optimizing stock portfolios for financial institutions. By processing many possibilities simultaneously, quantum computers could arrive at the optimal solution much faster.
4. Climate Modeling and Environmental Science
Quantum computers like Google Sycamore Quantum Computer could improve the accuracy of climate models, leading to better predictions of future climate scenarios. This could help policymakers make more informed decisions about how to address climate change. Additionally, quantum computing could aid in the design of more efficient energy sources, such as solar cells or batteries.
5. Artificial Intelligence and Machine Learning
Machine learning algorithms could see significant performance boosts from quantum computing, especially for tasks that require processing vast amounts of data. Quantum computers could speed up the training of AI models, making them more accurate and efficient.
Criticisms and Controversies Around Quantum Supremacy
While Google’s claim of quantum supremacy was widely celebrated, it also faced criticism. Some experts argued that the specific problem solved by Sycamore was not particularly useful in real-world applications. They contended that “quantum supremacy” is more of a symbolic achievement than a practical one.
For instance, IBM contested Google’s claim, suggesting that with a different algorithm and more optimized use of Summit, the same task could be completed in a matter of days, not thousands of years. Nonetheless, Google’s experiment still showcased the potential of quantum computing and set a benchmark for future research.
The Road Ahead for Sycamore and Quantum Computing
Quantum computing is still in its infancy, but companies like Google, IBM, and Microsoft, as well as governments and research institutions worldwide, are investing heavily in the technology. Here are some key areas of future development:
1. Building a Fault-Tolerant Quantum Computer
The ultimate goal is to build a fault-tolerant quantum computer—a machine that can perform long computations with minimal errors. This will require advances in quantum error correction, qubit stability, and scaling.
2. Developing Quantum Algorithms for Practical Use
Researchers are working on developing more quantum algorithms that can solve practical problems in fields like chemistry, physics, and computer science. This involves rethinking how we approach computational problems to take full advantage of quantum mechanics.
3. Integrating Quantum Computers with Classical Systems
For the foreseeable future, quantum computers will not replace classical computers but will work alongside them. Hybrid systems that combine classical and quantum computing will be crucial for solving problems that neither type of computer can handle alone.
Also read: Cloud Computing: Navigating the Skies of Technological Innovation
Conclusion
The Google Sycamore quantum computer marks a transformative moment in the field of computing. With its demonstration of quantum supremacy, it opens the door to a future where quantum computers can tackle problems that are currently unsolvable. While challenges like qubit stability, error correction, and scaling still need to be addressed, the potential applications in medicine, cryptography, climate science, and artificial intelligence make quantum computing one of the most exciting fields to watch.
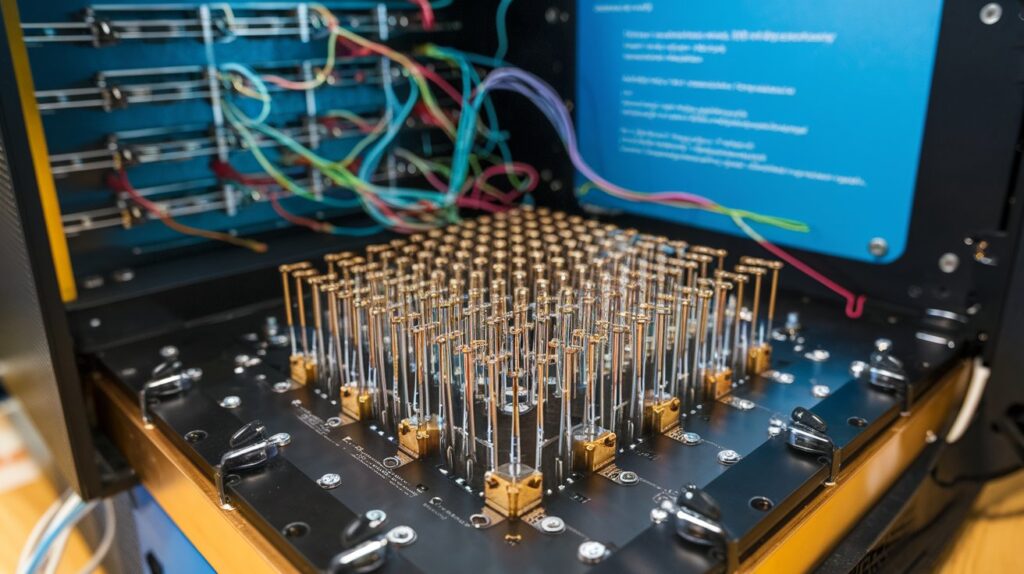
Sycamore has set the stage for future innovations, and as research progresses, the line between science fiction and science reality continues to blur. Quantum computing is not just a technological evolution; it could be a revolution that changes how we understand and solve the world’s most complex problems.
Frequently Asked Questions
1. Is Sycamore a real quantum computer?
Yes, Sycamore is a real quantum computer developed by Google. It was designed to demonstrate the principles of quantum computing and achieve quantum supremacy, meaning it can perform certain calculations faster than classical computers.
2. What is Google’s quantum computer Sycamore?
Google Sycamore quantum computer is a superconducting quantum processor with 54 qubits (53 operational). It gained attention for achieving quantum supremacy in 2019 by completing a complex calculation in just 200 seconds—an achievement deemed infeasible for traditional supercomputers, which would take thousands of years for the same task.
3. How powerful is Google’s quantum computer?
Google Sycamore quantum computer is powerful due to its ability to leverage quantum mechanics, enabling it to perform parallel computations across multiple qubits. While it’s difficult to quantify its power directly, its achievement of quantum supremacy indicates that it can solve specific problems much faster than even the most advanced classical computers.
4. How powerful is Sycamore?
Sycamore’s power lies in its ability to handle complex calculations through quantum operations. The specific task it performed involved random circuit sampling, which showcased its capability to outperform classical systems. This breakthrough is a stepping stone toward practical applications, but Sycamore is still in the experimental phase.
5. How fast is Google Sycamore?
Google Sycamore Quantum Computer completed its quantum supremacy experiment in just 200 seconds. This speed translates to solving a problem that would take a classical supercomputer approximately 10,000 years. This significant difference highlights the potential speed advantages of quantum computing.
6. Does NASA have a quantum computer?
Yes, NASA is exploring quantum computing technologies and has been involved in research partnerships to advance the field. NASA has collaborated with various organizations, including IBM, to leverage quantum computing for complex simulations and problems in aerospace, climate modeling, and more. NASA’s interest lies in utilizing quantum computers to enhance computational capabilities for various scientific missions.